3D Printing: The Unsung Hero of Neuroimaging Studies
3D printing has advanced rapidly in recent years. Whereas once the technology was suitable mostly only for prototyping and other applications that did not require high precision or repeatability, today it is widely used for the production of parts across a number of sectors. Not least of these is the biomedical imaging arena, where 3D printing is enabling quick, affordable fabrication of components for an array of imaging devices. In the following pages, researchers in the Neurophotonics Center at Boston University describe several projects that are benefitting from use of the technology, progressing in ways that otherwise might not have been possible.

Studying birdsong with 3D-printed miniature microscopes
Neuroscientists are increasingly interested in imaging animals’ brains as they go about their daily business— playing, exploring their environment, interacting with other animals. Studying these types of behaviors can reveal information about the brain that likely would not be accessible if the animals were constrained for use with conventional microscopes. To this end, companies have introduced miniature microscopes that, because of their size and mobility, enable monitoring of freely behaving animals in a range of different contexts.
At BU, researcher Ian Davison and colleagues wanted to study birdsong in the small, boisterous zebra finch, so they could better understand learning-related changes in the relevant region of the brain. Because most zebra finches will not sing if they are constrained, the researchers turned to miniature microscopes, borrowing a couple of commercially available instruments from other groups in the Department of Biomedical Engineering. They found, though, that these did not offer all of the features they were looking for, and the instruments’ closed designs meant they couldn’t tweak them as needed.
Enter 3D printing.
The investigators decided they could fabricate a microscope with the specs they wanted using a commercially available, consumer-grade desktop 3D printer. After surveying a number of instruments they selected the Formlabs Form 2 stereolithography 3D printer. With the Form 2, they would be able to print components with exceptionally small feature size (25 μm layers, 140 μm laser spot size), thus enabling printing of the high-resolution threads they would need to adjust the focal length of the microscope. For the 3D printing resins, they tested a number of resin types for light-blocking capacity, minimum print resolution, and auto-fluorescence in response to imaging wavelengths and chose FGPBLK01 and FGPBLK02. The final design incorporated the 3D-printed components and inexpensive off-the-shelf electronics.
Other options were available for the fabrication of the miniature microscope—including milling and other, related processes—but 3D printing offered significant time and cost savings over these. Using the technology, “an optical engineer can design an optical pathway and have a finished product in a couple of days,” says Daniel Leman, a researcher in Davison’s group at BU and one of the developers of the miniature microscope designed for the birdsong study. “To be able to do all of that inhouse is really incredible.”
Ultimately, the advantages of 3D printing for the fabrication of the miniature microscope extend well beyond the study of birdsong. It could enable advances in wearable technologies, generally, advances that could open up a range of new applications—including, says Davison, the study of neurochemical processes underlying various social behaviors in rodents.
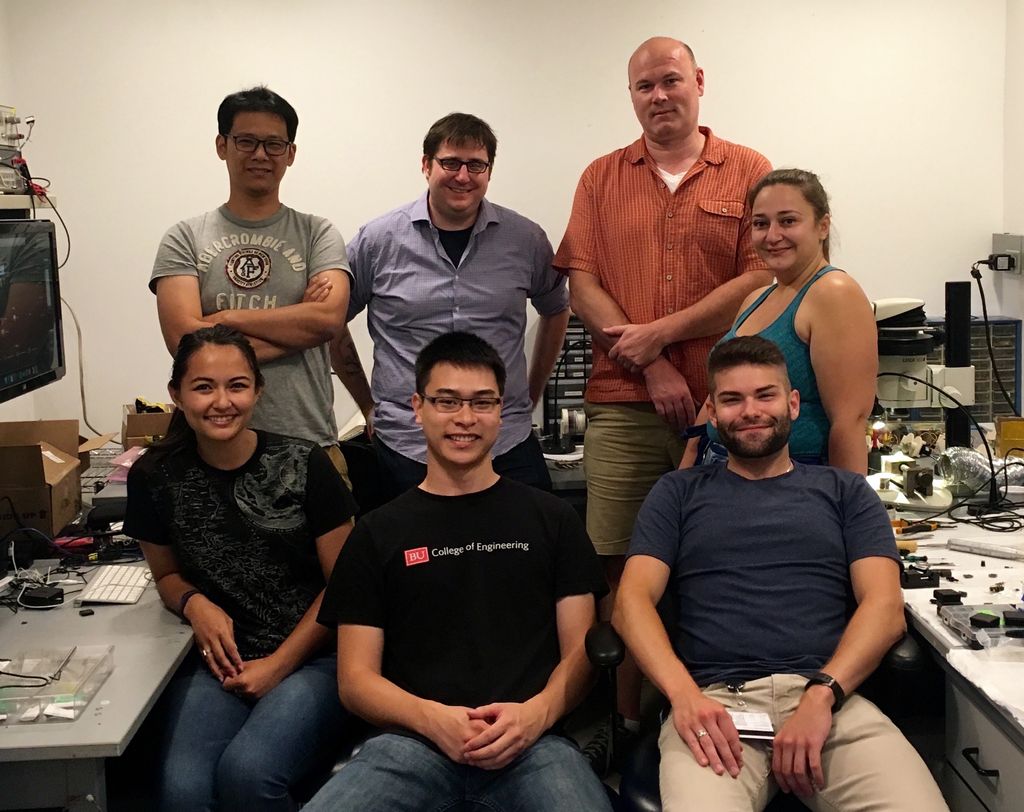
Advances could enable 3D printing on smaller scales and with complex materials
3D printing can be considerably less expensive and less time-consuming than other available processes. Just as importantly, though, it can enable fabrication of shapes that in many cases would be impossible to produce otherwise. “You can mold shapes and crevices and tolerances in 3D that you could never actually make with milling,” says Leman. “Many of the designs we have produced for the miniscope thus far would be frankly unfeasible using traditional techniques.”
And they have done so using commercial, off-the-shelf 3D printers. Timothy Otchy, a researcher in the Biology Department at BU, is developing an approach that will facilitate 3D printing on much smaller scales. The approach takes advantage of a technique called two-photon polymerization, where a pulsed laser is focused down to a tiny spot and swept through a liquid polymer in three dimensions, essentially writing the geometry of the desired structure in the polymer.
The approach could open the door to a number of new applications for 3D printing, Otchy says. “The off-the-shelf system we use to print the miniscopes is wonderful, and relatively inexpensive to get up and running, but the tolerances it can hold are probably, at best, submillimeter—maybe 250 microns, depending on the post-processing steps. A lot of the things we’re interested in printing—implantation devices, microlenses, other optical components requiring highly smooth surfaces—call for tolerances on the submicron scale.” Right now, he and colleagues are mostly using the new approach to print mechanical components like those found in the miniscope, but they plan to start exploring the other possibilities this year.
At the same time, they are exploring ways to incorporate complex materials into 3D printing. Currently, printing is only possible with a single material—acrylic, polymer, in some cases even metal. Being able to fabricate multi-material objects in a single step—patterning polymers and metals together, for example—could prove tremendously useful, allowing the researchers to interleave conductive materials into optodes or other optical elements.
3D printing caps for functional imaging of the human brain
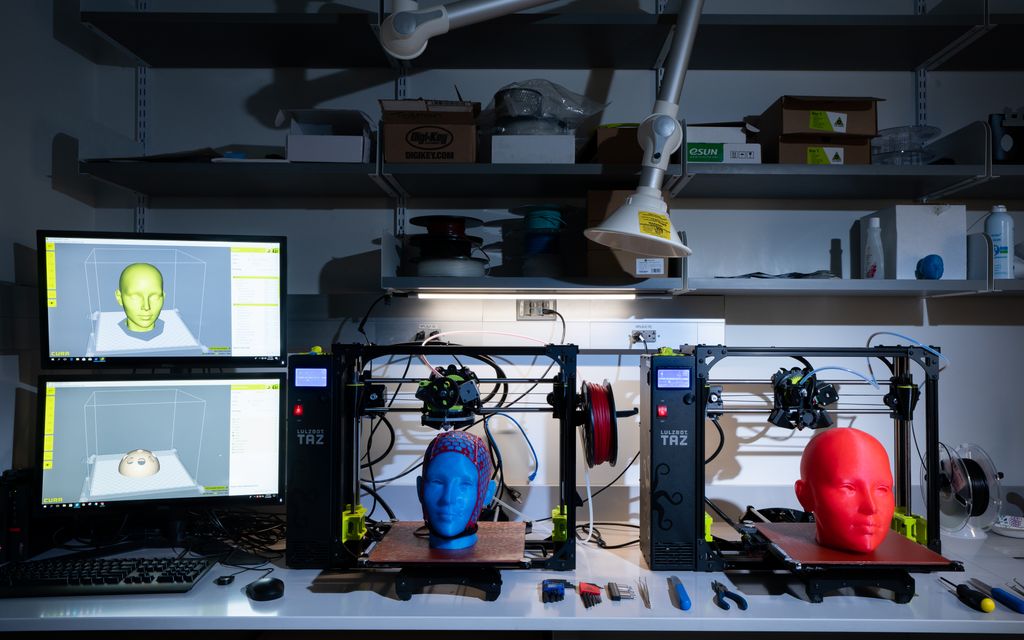
Elsewhere on the BU campus, researchers are working with 3D printing on a very different scale, using it to produce caps to hold imaging probes on the heads of human subjects.
The neuroimaging technique functional near-infrared spectroscopy (fNIRS) helps to shed light on the neural underpinnings of brain function by monitoring cerebral oxygenation—a proxy of neural activity—by transmitting near-infrared light into the head and monitoring it as it emerges. For many years, investigators have relied on plastic caps developed for EEG applications to position the light sources and detectors on subjects’ heads, punching new holes in the caps to accommodate the placement. But the most widely used caps—known as Easy Caps—are far from ideal for use with fNIRS.
“The thing is,” says Antonio Ortega Martinez, a graduate student in the BU Department of Biomedical Engineering, “the probe location is different depending on the experiment. It can be cumbersome making holes in caps all the time, especially if you want to have several caps for the same experiment. The holes might be inconsistent across the caps.”
Early last year, as part of a BME Senior Design project, undergraduate students Wesley Rivera, Erin Landry, Jessica Chou, Nikita Bhatia and Dale Tollman produced a design for an fNIRS cap that could be fabricated on demand using 3D printing. Here, the researcher needs only to tell the fNIRS-specific software package what area of the brain he or she wants to measure and the software calculates the position of the probes on the cap and sends the design to the printer. Not only does this enable customization of the probe’s location, it allows researchers to specify the size of the head for which they are making the cap. Being able to tailor caps to fit subjects’ heads is especially important because so many fNIRS investigators work with infant populations as well as adults.
The initial design and tests of the fNIRS cap were done by Zack Starkweather at the MGH Martinos Center for Biomedical Imaging, a collaborating Institution with the BU Neurophotonics Center. Other contributors to the project at BU include Cameron Snow, Nathan Perkins and Alexander Lühmann, as well as Antonio Ortega Martinez.
Have you found exciting new uses for 3D printing in neurophotonics-related applications? Let us know by emailing David Boas and Elisa Ronchi.