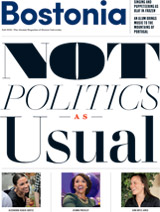
Bostonia is published in print three times a year and updated weekly on the web.
For 100 years, doctors have treated some broken bones by tugging on them. It’s true.
Millions of people break bones every year—in this country alone, there are close to eight million fractures annually —and of these, 5 percent to 10 percent fail to heal. The medical bills for bone repair clock in at an estimated $70 billion.
Fortunately, bones can regenerate, and orthopedic surgeons can enhance this regeneration with a process called distraction osteogenesis. First, the bone is surgically broken, pins are inserted above and below the bone incision, and then a metal device is attached to the pins. The device slowly cranks the bone apart by only millimeters a day, and over time, new bone grows from both ends of the fracture, filling the gap.
While the procedure can increase a person’s height (think Ethan Hawke’s character in the film Gattaca), it can serve many other purposes, including lengthening a single bone that is abnormally short because of a childhood injury and lengthening and reshaping bones to help correct congenital deformities. The success of distraction osteogenesis in these cases has also led doctors to use it to treat a bone fracture that has not healed well.
One of the more interesting things about distraction osteogenesis is that despite decades of observation doctors and researchers still don’t know exactly how it works. Elise Morgan a College of Engineering associate professor of mechanical engineering and of biomedical engineering, hopes to find out, and she is honing in on the conundrum in a series of related research projects.
The director of ENG’s Orthopaedic and Developmental Biomechanics Laboratory, she believes that a better understanding of the bone regenerative process could lead to noninvasive ways to examine fractures and less invasive ways to treat them. It could also help orthopedic surgeons select treatment parameters based on the type of bone, the type of patient, and the device that is used.
“Our tissues and cells are very responsive to the mechanical forces that they experience,” says Morgan, who was awarded the 2013 Kappa Delta Young Investigator Award by the American Association of Orthopaedic Surgeons and named a 2012 College of Engineering Distinguished Faculty Fellow. “So the stresses we put on our bones as we sit, walk, and jump are sensed by our tissues and have an effect on how they behave and ultimately heal. Understanding this process is critical in understanding why some bone injuries heal, and some don’t.”
Composed mostly of minerals and protein—mainly collagen—bone has a complicated structure that is sometimes likened to a coral reef, because of its porous structure, strength, and ability to regenerate. This regenerative capacity is something that bone cells share with liver cells. Better ways to harness this regenerative capacity are sorely needed: bone is the second most frequently transplanted tissue, after blood.
Just as weight lifting strengthens muscles because of the trauma to the muscle fibers, a force applied to a bone can change its structure. A biomedical theory known as Wolff’s law asserts that in response to increasing applied force—called loading—bone will remodel itself to support and accommodate that loading.
Although distraction osteogenesis is widely used, the procedure has a 30 percent complication rate. Doctors and researchers can measure how many millimeters the bone has been stretched, but they don’t know much about how that stretching ends up loading the fragile new tissues growing with the gap or ends up affecting how stiff and strong those tissues become.
To tackle these unknowns, Morgan has collaborated on various studies with Thomas Einhorn, a School of Medicine professor of orthopedic surgery, biochemistry, and biomedical engineering and chair of orthopedic surgery and chief of orthopedic surgery at Boston Medical Center, and Louis Gerstenfeld, a School of Dental Medicine research professor of biochemistry, as well as with students in Morgan’s lab.
Previous studies had looked only at how much the gap in between the two pieces of bone was lengthened and tried to correlate that amount to how stiff and strong the lengthened bone became. Other studies tried to determine what kind of loading microenvironment in the gap would aid the healing, but used only computer simulations that hadn’t yet been proven to give accurate results. The problem, Morgan decided, was that there were no measurements of the loading microenvironment and therefore no way of relating this microenvironment to how much bone regeneration occurred. In short, there was not yet a reliable way to predict what the healing process would be like.
Researchers in Morgan’s lab “broke,” or cut, bones and twice a day they lengthened that gap between the severed bone pieces, monitoring changes with CT scans. They measured the strains, or deformations, caused by the lengthening, and what they found supported earlier suspicions that not all of the tissues in the gap experience the same amount of strain. They also found evidence that bone cells’ behavior is influenced by the type of loading microenvironment: whether the new bone tissue is stretched or sheared. Morgan compared mild amounts of lengthening to larger amounts, and found no simple relationship when it came to healing. Consequently, she says, there is no one-size-fits-all procedure for distraction osteogenesis to guarantee that a bone will heal successfully.
Next the researchers compared the mechanical environment in distraction osteogenesis to that in the much less favorable result of pseudarthrosis, or false joint. A pseudarthrosis can form at the site of a bone fracture when that fracture doesn’t heal.
Instead of pulling the bone cleanly apart as is done in distraction osteogenesis, the researchers applied a bending motion, which resulted in formation of a pseudarthrosis. The two motions, they learned, created very different mechanical environments, which likely explained why the amount of healing was so different. The comparison also served to provide more evidence that whether and how much new bone tissue is stretched or sheared during healing has a major influence on how much more bone subsequently forms.
Finally, Morgan worked with Mark Grinstaff, a College of Arts & Sciences professor of chemistry and an ENG professor of biomedical engineering, to develop a method using CT scans that can reveal the formation of cartilage in fractures. Most bone fractures heal by forming cartilage and then bone, so knowing that cartilage has formed early on in a fracture is an indication that the healing is going well.
“Whereas previously we wouldn’t know how the healing is progressing until someone’s bone is about halfway to being healed, this new CT scan could let us come in a quarter way through to see if the healing is progressing normally,” Morgan says. “No cartilage means you probably won’t get adequate formation of bone and won’t have a well-healed fracture. Doctors could intervene much earlier.”
She is still studying what goes on at the bone’s molecular level, and what kind of molecular mechanisms are activated by the mechanical environments that produce bone or cartilage. “We’re interested in how a certain type of mechanical loading—for instance, the stretching that is applied in distraction osteogenesis—might put into motion some of those molecular mechanisms that then are responsible for the healing process,” says Morgan, who is also looking at the mechanisms at a genetic level. She believes she has identified some proteins that are active in bone healing and plans to work to find the mechanical microenvironments that can trigger them.
“When a bone fails, that has a big impact on someone’s quality of life,” Morgan says. “The mechanical engineering concepts we use define how much strength and stiffness this bone has regained and whether that regaining is enough to restore quality of life. As doctors and scientists, it’s our job to study how we can get bones to heal faster and with fewer implications.”
Related Stories
Bonecrusher
Engineering professor Elise Morgan looks for a better way to predict spine fractures
Bone Detective
Understanding bone weathering may help determine time of death
Two from BU Cited for Encouraging Women in STEM
Lauded by magazine for their inspiration
Post Your Comment