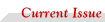 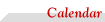 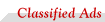 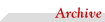
Mailing
List
Contact
Us
Staff
|
 |
Nanoscience
on the boundaries
Unlocking the secrets of living cells
By
David J. Craig
 |
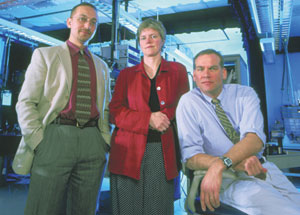
|
|
Selim Ünlü, an ENG professor of electrical
and computer engineering (left), Anna Swan, an ENG research assistant
professor
of electrical and computer engineering, and Bennett Goldberg, a
CAS professor of physics, share a lab in the Photonics Center,
where they conduct interdisciplinary research in nanoscience. Photo
by Kalman Zabarsky
|
|
 |
By developing new microscopy techniques that peer deep inside living
cells, an interdisciplinary team of BU scientists and engineers soon
could enable medical researchers to better understand the subcellular
processes in pathogens such as E. coli, salmonella, and shigella.
The
innovative subcellular imaging project is led by Bennett Goldberg, a
CAS physics professor, and Selim Ünlü, an ENG professor of
electrical and computer engineering. Goldberg and Ünlü, who
share a laboratory at the BU Photonics Center, are experts in nano-optics,
a field that uses light in new ways to see tiny objects in ever-finer
detail.
In 2002, their research team received a $1.7 million grant from
the National Institutes of Health to develop a technique called high-resolution
spectral
self-interference fluorescence microscopy, which has the potential to
produce images of biological phenomena where points as close as a few
nanometers apart can be clearly differentiated. Currently, the researchers
are developing methods for observing the structure of the dysentery-causing
shigella bacteria, showing how proteins and other molecules within shigella
cells go about their work.
Fluorescence microscopy, one of the primary
tools for probing biological systems, involves imaging molecules that
emit light when excited by an
outside source, like a fluorescent watch dial. These fluorescent molecules,
or fluorophores, can occur naturally within cells or be introduced into
cells. At present, the technique allows researchers to see things as
small as 300 nanometers (a nanometer is one billionth of a meter, or
about 1,000 times smaller than the width of a human hair). “That
sounds like a short distance, but there are many processes inside cells
that happen at much smaller length scales,” says Goldberg. “We
want to bring that resolution down to 10 or 20 nanometers so we can observe
things like transmembrane activity, where the membranes may be only 15
nanometers thick, and activities in the cell where multiple proteins
may be working very closely together.”
Existing instruments such
as electron microscopes already can render images of biological features
at resolutions of just a few nanometers.
The problem with that technique, Goldberg explains, is that it requires
killing the cell, freezing it, and slicing it thinly before bombarding
it with high-energy electrons, which damage the sample as they bounce
off it. His goal is to develop an instrument that can locate, in real
time and three-dimensional space, the precise position of certain proteins
in living bacteria and viruses.
A key innovation of Goldberg’s and Ünlü’s
technique is that unlike standard fluorescent microscopy, which uses
a single lens
to collect light emitted in one direction, it uses an additional lens
or mirror to collect light emitted from fluorescent particles in both
upward and downward directions. The way these light emissions interfere
with each other provides a new level of accuracy about the location of
the fluorescent particles and their actions within the cell.
“
Fluorescence microscopy is an old technique that we’re using in
a new way,” says Goldberg. “The trick is figuring out how
to view multiple fluorophores in one area, and to understand in spatial
terms what you see in the spectral domain.”
That’s one of
the technical challenges Goldberg and Ünlü are
tackling with Clem Karl, an ENG professor of electrical and computer
engineering, and Anna Swan, an ENG research assistant professor of electrical
and computer engineering. The team also is collaborating with researchers
at Massachusetts General Hospital who have expertise in the biological
structures of shigella bacteria and similar pathogens. Such cross-pollination
among disciplines, Goldberg says, is essential to solving problems in
nanoscience, which involves the study of phenomena at the atomic and
molecular levels.
“
The real breakthroughs in nanoscience are going to happen at the boundaries
between disciplines,” says Goldberg, who with Ünlü formed
the BU Nanoscience Working Group in 2002 to encourage physicists, chemists,
biologists, engineers, and computer scientists working in nanoscience
to collaborate, share lab space, and jointly fund postdocs. Currently
they are preparing to form a center for nanoscience research at BU.
“
It’s pretty unusual for a condensed-matter physicist like myself
to have an NIH grant to do biological imaging,” Goldberg says. “My
expertise extends up to the edge of molecular biology, and that’s
where I look to collaborators who can help develop the biological model
systems to test a new microscopy, and who know the critical biological
questions to ask.”
Interdisciplinary nanoscience research at BU
has been given a boost in recent years, he says, by the dramatic growth
of ENG’s biomedical
engineering department, which in 2001 received a $14 million Whitaker
Leadership Development Award (see related story on page 3). “The
strength of ENG’s biomedical engineering department is part of
what makes Boston University uniquely suited for this type of research,
because researchers doing material and device-level science in areas
like physics and engineering have colleagues they can talk to who understand
biological applications,” Goldberg says. “The department’s
enormous growth, and all the work being done there on drug delivery,
tissue engineering, and other human physiology applications, is going
to make a big difference in the success of nanoscience research at BU.” For
more information about Goldberg’s and Ünlü’s research,
visit ultra.bu.edu.
|
 |