Can Droplets be Used to Stop, Instead of Spread, Disease?
ENG, CDC researchers quantify how droplet formation might damage microbes, reducing disease transmission
By Patrick L. Kennedy
It happens in a flash. As you cough up a thread of the fluid that lines your respiratory tract, it breaks into tiny droplets, as small as a micrometer in diameter. Some of those droplets, or aerosols, might hang in the air for minutes or even hours. And some of them might contain pathogenic microbes—bacteria or viruses that cause disease.
What if you could slow down that moment when the droplets are formed? That’s what researchers from the College of Engineering and the Centers for Disease Control did in a study—recently published in Physical Review Fluids—on the violent, unseen forces unleashed during aerosol formation. Their findings have bearing on the transmission of respiratory diseases such as COVID-19.
“These droplets do contain biological life,” explains Mechanical Engineering postdoctoral associate Oliver McRae, the study’s lead author, and to spread disease, “that life must be able to survive this whole churning and agitation process.”
The agitation occurs as droplets break off from a liquid thread to go spinning into the air, where someone might inhale them. But, paradoxically, the same churning process that gives the aerosol-borne microbes a chance to spread can also stress and kill them before they get that chance.
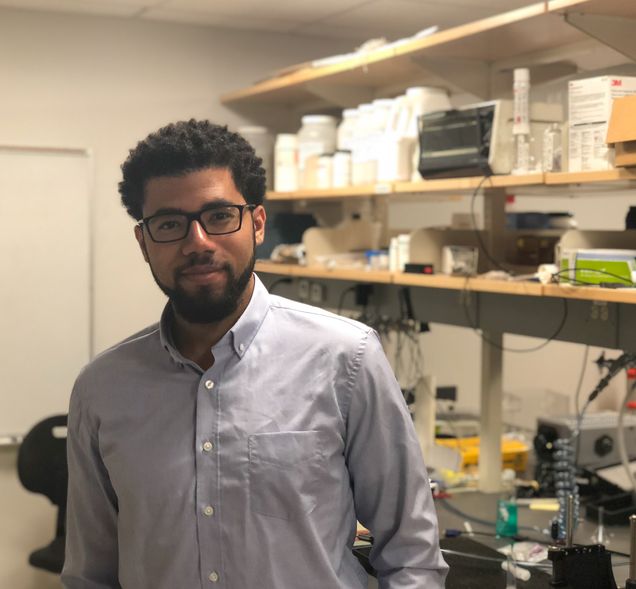
“Imagine you have a fish in a bucket of water,” says McRae. “And now you’re stirring that water faster and faster. At some point, that fish is not likely to survive. That’s kind of what we have in the droplet, albeit on a much smaller scale.”
McRae and Associate Professor James C. Bird (ME, MSE) studied the droplet formation process in Boston University’s Fluid Lab. They shot extremely fine threads of water through the air and recorded the motion with a high-speed camera capturing up to 350,000 frames per second.
When they played back the microsecond-by-microsecond images, McRae and Bird witnessed the dynamics of the “pinch-off,” when the droplets break from the thread. The droplets’ movements confirmed the stressors calculated by the researchers’ computer simulations of the churning action that might wreak havoc on microbes.
The computer simulations showed the stressors to be highly sensitive to the droplet size: The smaller the droplet size, the greater the stressors. “The stressors increase two-and-a-half orders of magnitude for every order of magnitude decrease in droplet size,” says McRae.
Some previous studies touched on hydrodynamic agitation as a factor in microbial transmission, but McRae and Bird’s study—done in collaboration with a colleague at the CDC—is the first to quantify it, and it adds to the scientific community’s knowledge of how disease spreads.
While agitation does not kill all microbes, the research advances the tantalizing possibility that it could be wielded deliberately to tamp down their spread. Earlier research established that saline spray increases surface tension on droplets, which, McRae says, could make the agitation stronger. McRae—who earned doctoral and master’s degrees in Mechanical Engineering from ENG in 2019 and 2016, respectively, and a bachelor’s in biology from the College of Arts & Sciences in 2012—is planning to pursue this research further.
“If you can lower the number of viable pathogens in aerosols, then you can lower the infectious load and reduce the likelihood of transmission,” says McRae.
“What I like about this work is that I’m able to combine my undergrad and grad training,” McRae says. “I get very excited about the possibility of being able to help people with reducing transmission and reducing infections.”
In addition to Physical Review Fluids, McRae’s paper will appear in the American Physical Society’s special collection of COVID-relevant research.