Scientist Profile – Professor Christopher Chen
Christopher Chen, M.D., Ph.D.
Professor of Biomedical Engineering
Principal Investigator for the Tissue Microfabrication Laboratory
Director of the Biological Design Center
Professor Christopher Chen heads the Tissue Microfabrication Laboratory which seeks to understand how adhesive, mechanical, and biochemical interactions drive cell and tissue function, to use this knowledge to build biomimetic tissues as experimental models, and to direct tissue remodeling and regeneration.
Professor Chen reflected on the challenges of engineering functional biological tissue, the “shape acrobatics” behind the formation of our tiniest blood vessels, and the sports injury that first catalyzed his journey toward the study of tissue microfabrication.
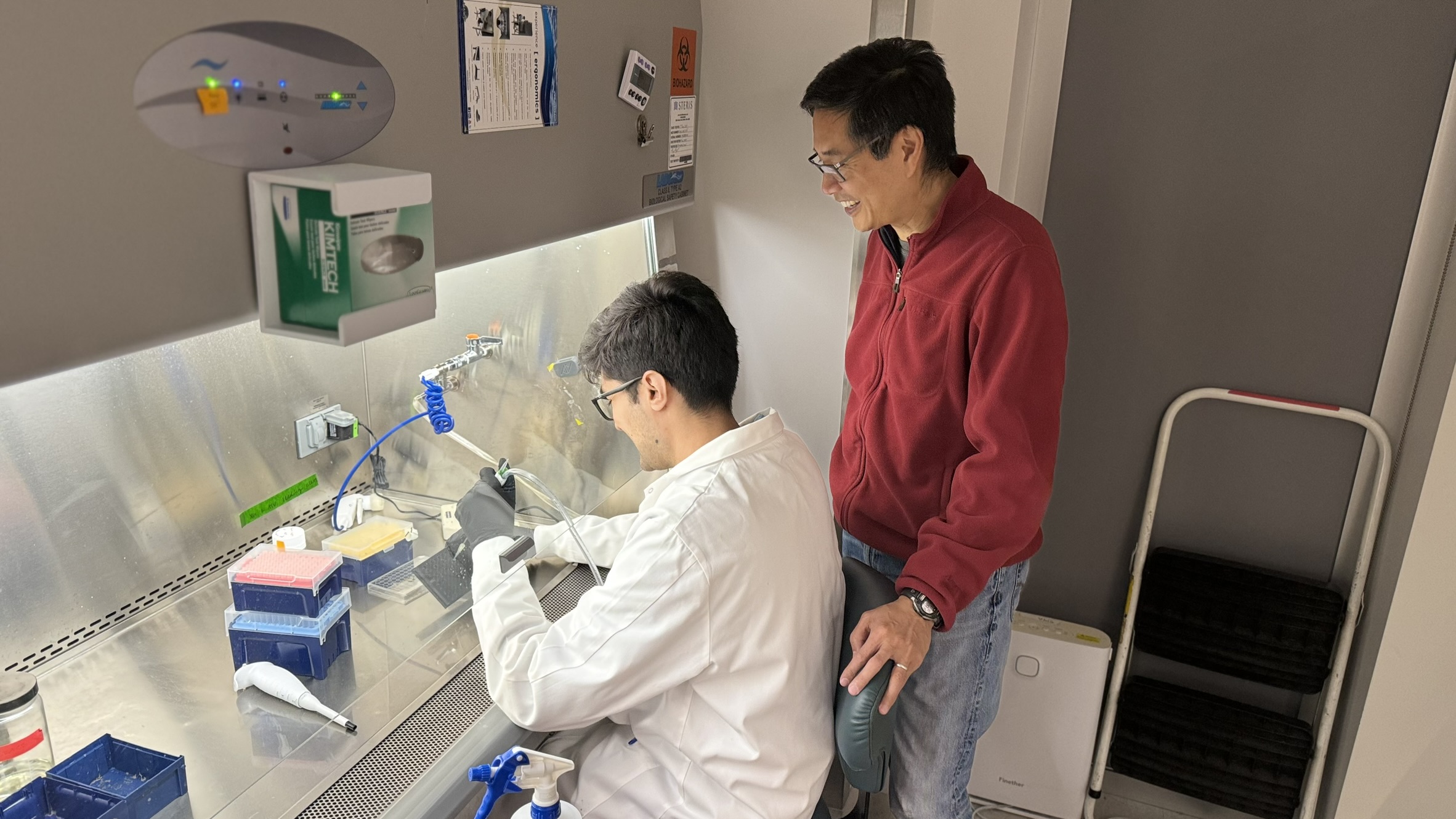
What is the focus of your lab?
My lab aims to understand how cells organize into tissues and how to control that process to engineer synthetic tissues, whether that’s heart tissue or liver tissue or what have you. What are the control systems that enable cells to organize in a particular pattern? Can we encourage cells to make the kinds of tissues that we want? And can we use those engineered tissues to study diseases that right now we can only study in animals or in people?
Can you describe a representative study that explores these questions?
We’re currently working on how to build blood vessels—not large blood vessels, but those small, capillary blood vessels that permeate throughout all our tissues facilitating the delivery of oxygen and nutrients via the flow of blood. We want to understand how the cells that line blood vessels, which are called endothelial cells, construct these web-like networks of tubes, called lumens, that blood can flow through. This transition from cells that just kind of hold onto each other to the formation of open tube-like structures through which blood can flow is, to me, just an amazing process of shape acrobatics.
Why is this capillary network useful? Well, right now, we can take a cardiac cell or a liver cell and organize hundreds of them into small tissue-like units that behave similarly to how they do in our body, but the structures are very tiny, less than one millimeter. If we then try to expand that and build a centimeter-scale structure, what happens is that all the cells in the middle of that tissue die because there’s no way to get oxygen to them. So building that capillary vessel network creates the plumbing that can deliver nutrients and oxygen throughout that mass. The challenge of engineering a vasculature that can support tissues has yet to be solved, but when it is solved, the medical and therapeutic applications will be significant. I’m interested in those applications, of course, but also still just fascinated by the basic scientific question, “How the heck do the cells do it?”
What’s an example of something you learned recently from your studies of vasculature?
One thing we’ve learned concerns the material in which the cells are sitting, known as the extracellular matrix. We have learned that the composition, density, and stiffness of that material all impact how these cells behave and enable them to form these structures. We also know that there are lots of other cues that cells pay attention to. For instance, when fluid flows through the tissue, it acts as a trigger causing the cells to form these tube-like structures. And once the vasculature is established, the flow also impacts the health of the cells themselves. These cells have evolved the ability to sense what’s known as the “shear stress,” or the mechanical shearing of fluid flow over them, and the level of that stress impacts, for example, whether the vessels become more permeable or more tight, more anti-inflammatory or pro-inflammatory. Now we’re trying to better understand how to use flow as a mechanical cue to guide the formation of vessels.
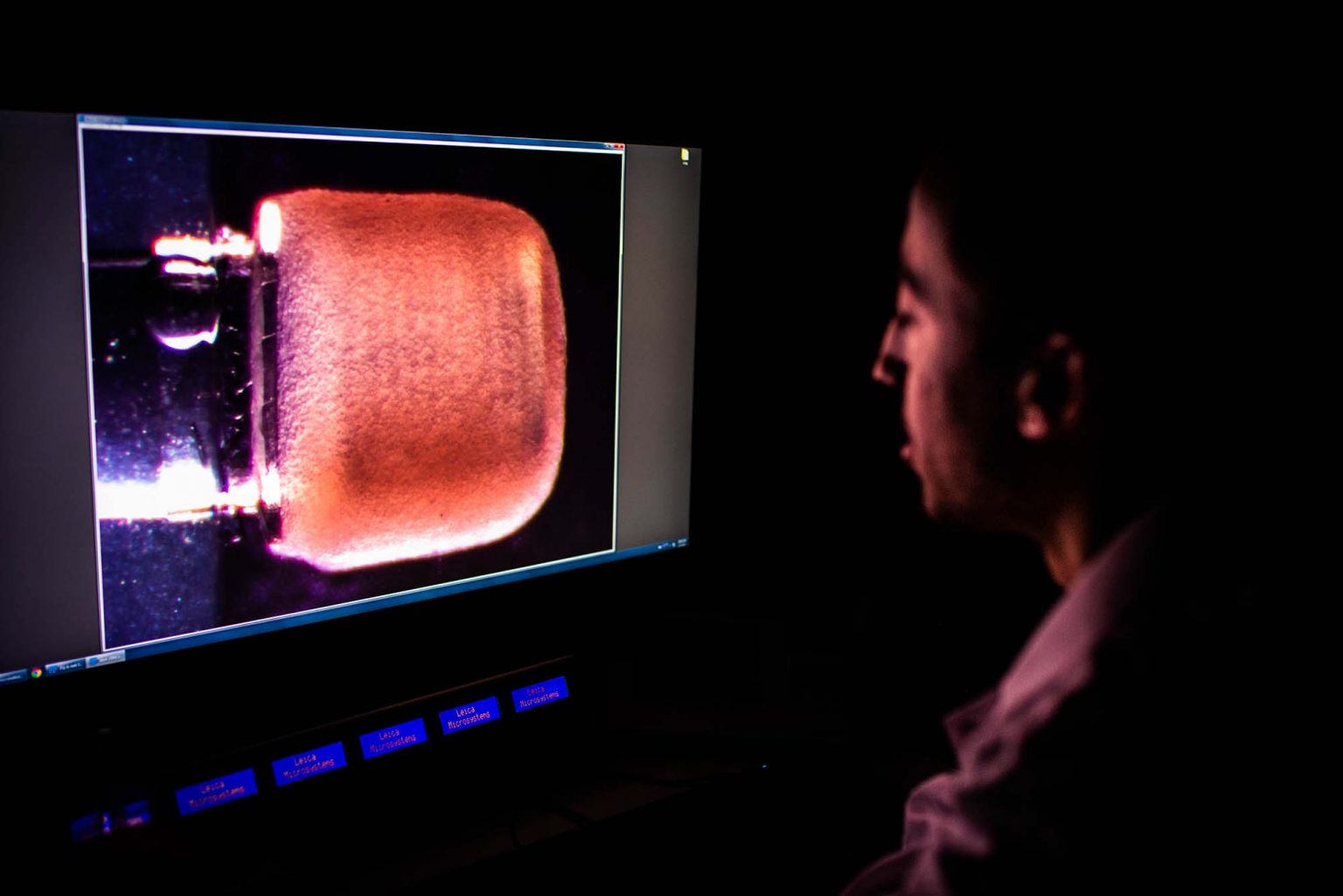
What else besides vasculature are you studying in your lab?
We’ve been doing a lot of work on building heart tissue. We are part of this big grant involving many other investigators, both here at BU and in other places, that are trying to figure out how to take stem cells, differentiate them into cardiomyocytes—the cells responsible for heart contraction—and then assemble those cardiomyocytes into a multicellular tissue, an actual mass that we think of as muscle. And again, there are many different types of cues that are used to try to induce these cells to form muscle. Most of the field is focused on soluble cues, such as growth factors that can sort of turn these programs on. What we’ve focused on are electrical and mechanical cues. For instance, if the cardiac muscles experience resistance as they contract, the resistance actually triggers them to get stronger, not unlike skeletal muscle, and so we’re trying to study how that works.
How did you decide to focus on tissue microfabrication in your career?
I started my career interested in mathematics—I enjoyed the beauty of mathematical problems and trying to solve them—but I also did get interested in health issues early on, mostly in the context of sports injuries (I had sustained a knee injury as a runner). As an undergrad I ended up working in a lab at MIT that was trying to build artificial cartilage as a biomaterials problem, and what I quickly discovered was that all these material solutions to making joints would ultimately fail because, as with any material, when you continuously apply a mechanical load to them, they wear and tear and eventually fall apart. It was a frustrating realization. I learned that no matter what we tried, nothing was like living cartilage.
When you consider how many times a heart contracts or how many times a knee bends over the course of our lifetimes, these biological systems generally don’t fail (or at least not nearly as quickly) in part because they’re continuously repairing and turning over. When proteins get damaged, the cells express new protein to replace it. As I started my graduate career, I wanted to better understand how cells are able to continuously regenerate these tissues and materials.
What are your favorite activities outside of the lab?
I really love to hike. My wife and I spend a lot of time in the White Mountains of New Hampshire. I also loved coaching my children in soccer back when they were younger (now they are happy to be left to their own devices!).
What’s your favorite book?
I have to say I still love Tolkien’s Lord of the Rings.
What is your most desired superpower?
The power to stop time—then I’d have all the time in the world to finish and submit grant proposals! I also wouldn’t mind slowing down how fast my kids grow up.