Tim O’Shea’s Lab is Finding Avenues to Repair Neurotrauma
by Danny Giancioppo
Although Assistant Professor Tim O’Shea (BME, MSE) was always interested in STEM, it wasn’t until his junior year of high school that his interest evolved into a personal mission.
Growing up in Brisbane, Australia, there was a surplus of mining and mechanical engineering opportunities in the state, lending itself to a vibrant engineering community. However, when his best friend suffered a spinal cord injury playing rugby, O’Shea knew he wanted to study the Central Nervous System (CNS) to improve methods of rehabilitation and repair.
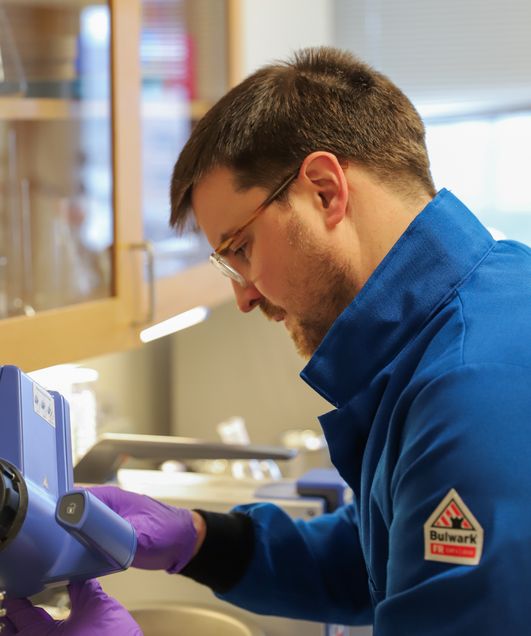
“He was an in-patient for almost a year,” O’Shea recalls. He would work with his friend in the process of rehabilitation, and was confronted at a young age with the harsh reality of living with a spinal cord injury. “[Later,] I had this serendipitous interaction with a professor at the university I ended up going to for undergraduate, who told me about medical engineering and the idea of developing exoskeletons and various prosthetic-type devices to help people walk again.” From there the mission was simple: O’Shea would go to university and pursue a degree in medical engineering, seeking to help people like his best friend following various neurotrauma.
During his time as an undergraduate, O’Shea was introduced to the widespread capability of cellular-molecular manipulations in biomedical engineering. Where he once sought to help with the treatment of spinal cord injuries, he now found there were methods of research which began carving a path toward tissue regeneration and ultimately recovery of spinal cord function. This led O’Shea to the U.S. for his PhD, where he’s continued his research ever since––for the last several years, at Boston University.
In the O’Shea lab, researchers advance the opportunity for spinal cord repair through biomedical engineering and research. “Not purely on spinal cord injury,” O’Shea adds, but other neurotrauma. “We work on stroke as well as neurodegenerative diseases such as Parkinson’s Disease,” primarily through their research on glial cells.
Glia literally means “glue,” and it’s an apt name for the cell-type’s purpose in neural tissue function. Glial cells, which are commonly sub-categorized as astrocytes, microglia and oligodendroglia, were once considered merely there to contribute to the mechanical integrity of the central nervous system, allowing the main functional cells, neurons, to direct movement and sensation. But this was an oversimplified understanding.
“[Glia] are critical to regulating neural circuit functions and ensuring the brain and spinal cord remain healthy,” O’Shea explains. “You can’t have brain or spinal cord tissue without glia.” Without glial cells, O’Shea goes on, the neurons are either doomed to die or functionally fail within the context of their role. “They’re critical to maintaining the viability of circuits, but not only do they do that, they essentially are the gatekeepers of the outside world to the CNS.”
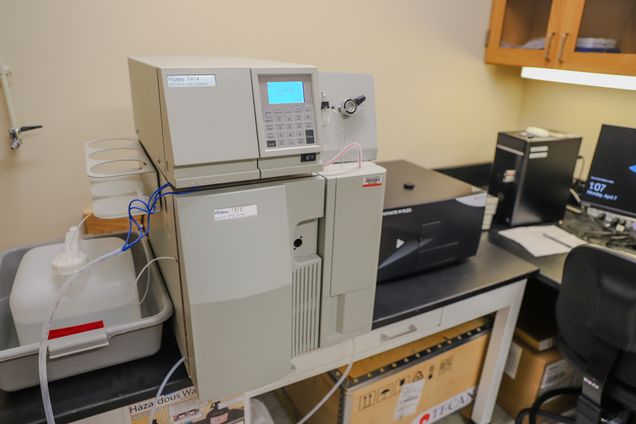
Glial cells are responsible for regulating other cells and molecules that may enter the CNS and partition the CNS apart from other cells and tissues. In this way, O’Shea views them as one of the most integral cell types in the brain––and this is within a healthy context. When it comes to perturbations, such as brain trauma or neurodegenerative disease, they are second-to-none. The glial cells will respond to these perturbations by undergoing adaptations to regulate repair and functionality of the brain, more so than neurons. This is why, in the O’Shea lab, they primarily focus on glial cells––a form of research that lends itself to convergent projects across the Neurophotonics Center, and University at large, which they break down into three categories of work.
“We work in three main initiatives,” O’Shea says. “CNS injury, neural interfaces, and glial drug delivery.”
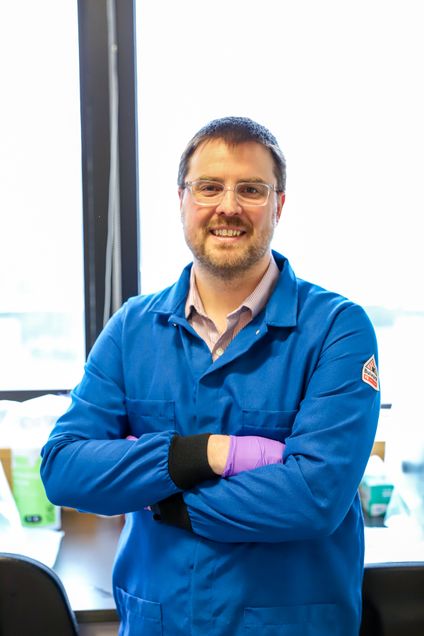
“We have projects focused on developing ways to better deliver therapies that have relevance for neurodegeneration––like Parkinson’s disease and Alzhemier’s disease. Obviously, our work in CNS injury is relevant to stroke, spinal cord injury, traumatic brain injury. And then the neural interface work––there’s more and more interest in putting electrodes into the brain without really thinking about the ramifications of that long-term for people’s brain health. If we can develop better ways to interface with the CNS, we can develop safer electrodes. All of those are really important for furthering potential clinical treatments.”
In their CNS injury work, the research leans toward modulating the function of endogenous glia for wound repair––using material systems to manipulate key features of wound responses such as metabolism or proliferative programs––to augment a neonatal-like recovery.
“In very young animals, they have these kind of ‘super’ glial cells that are able to mount a wound response more effectively. As a result, certain CNS injuries in very young mammals will naturally regenerate […] We lose that competency over the first couple weeks of postnatal development, and we instead rely on fibrotic wound healing.” Much of the lab’s efforts therefore aim to replicate neonate wound repair responses, while simultaneously hampering the adult wound responses, which are less effective.
“That’s endogenous cells––cells that are already in the brain and spinal cord,” O’Shea continues. “Another aspect of CNS injury work is in transplantation. How can we use an exogenous source of cells to do wound repair? We transplant cells that turn into glial cells, and we develop ways to enhance their survival and their function to do better wound repair in the context of these CNS injuries.”
One active example concerns organoid research, one of the widest cross-convergent research projects currently ongoing at Boston University. Organoids are literally “mini organs,” small, healthier cells placed onto a correspondingly damaged organ––such as the brain, through any number of neurodevelopmental or -degenerative ailments. When regulated properly, these miniature cells showed signs of reintegration with the damaged organ, providing it with new, functioning cells which could, in the best of cases, lead to organ repair and renewed functionality.
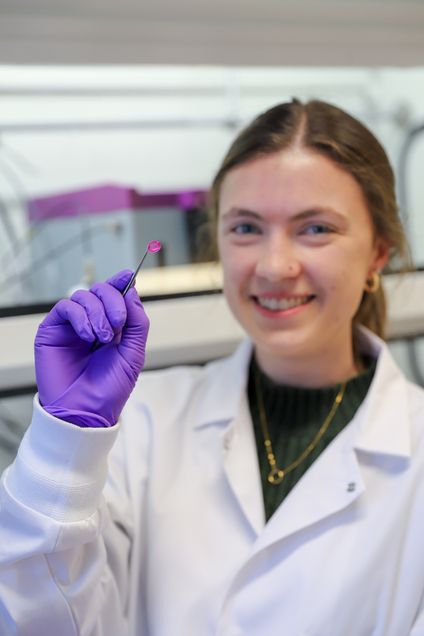
“The organoid project is kind of interesting,” O’Shea says. “We’re essentially using these material films that we can load with molecular regulators. We can place [them] on the surface of the brain, or over the top of the implanted organoid, to provide the developmental morphogens that direct the patterning of the organoid.” A consistent problem with organoid implantation is the aimless development process of the new cells. With no instruction, there can be a heterogeneous mass of cells––sometimes leading to tumor growth. The use of material films thus serves an “engineering approach,” substituting the developmental morphogen gradients which otherwise take place during development.
Ironically, this was only a byproduct of the original material goal. “We were trying to use [material films] to modulate the foreign body response around devices,” O’Shea says. The material was not only a film, then, but a coating around the perceived interface of electrodes and devices implanted into the CNS.
This serendipitous result has led to multiple collaborations across Boston University’s campus with other researchers forwarding organoid research, but it isn’t the only field that promotes convergence. Like many Neurophotonics Center labs, O’Shea’s group is an inherently interdisciplinary collection of scientists with several multifaceted projects. This has led to such collaborations as with the Boas lab, in their research on modulating glial function in strokes, and implanted device projects with the Economo lab––who have developed microprism models for neuron imaging––and integrating their materials onto the prism to make them optically transparent for imaging purposes.
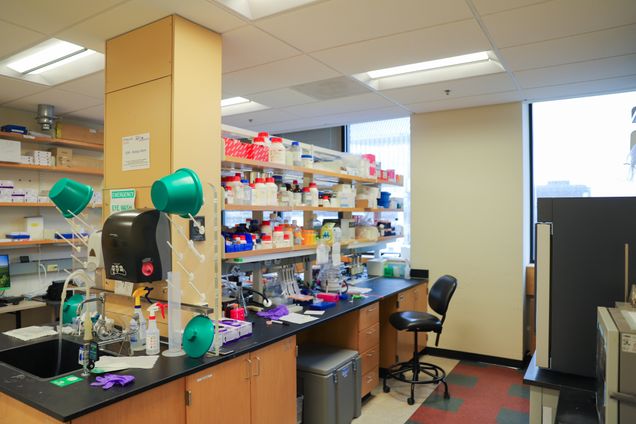
“We see ourselves as both a group that makes new material tools to do interesting biological studies,” O’Shea explains. “And we bring [faculty] along who have expertise in complementary areas––mostly optical tools––to be able to elevate the kind of studies that we can do.”
The same rule applies for his students, both new and returning: innovate first, and then apply widely. To O’Shea, a varied pool of expertise determines a successful lab space. “It’s not like we just have students that purely make new materials, although that is an aspect of people’s projects.” O’Shea explains that “those same people who are [making materials] are also studying their materials in vitro cell culture models, in in vivo animal models,” and that some students also have the complement of the optics element— intravital imaging.
Many of the projects that the O’Shea lab works on are largely supported by the NIH, spinal cord injury research foundations such as the Craig H Neilsen, Byron Riesch, Paralyzed Veterans of America, and Wings for Life, as well as the Neurophotonics Center. Currently, three R21 grants and an R35 MIRA, all from NIH, are providing the funding and resources necessary for the O’Shea lab to continue its research at a high level. In the current political environment, O’Shea feels it’s particularly vital to acknowledge and thank the US tax payers and the federal government workers for their assistance in enabling such important work. “That support is crucial to do the work. It doesn’t just happen in a vacuum. We can’t do our work without that kind of support.”
And the support of such institutions doesn’t only help fund the research, O’Shea adds. It also helps to improve the research itself. “A lot of these grants don’t get awarded the first time. So the process of going through peer reviews and study sections––it helps cement the idea, focus the idea, refine the idea to really hone in on what’s most important to go after. And these projects are tackling a really important question that is addressing a really important need.”