BU Takes On Cancer: Mapping Metastasis
Computer simulations model the spread of cancers

ENG’s Muhammad Zaman has spent the last decade trying to understand this peculiar hallmark of cancer: why and how do cancer cells move from one part of the body to another? Photo by Cydney Scott
In part four of a five-part series, BU Today examines the many ways BU researchers are working to demystify, treat, and prevent cancer.
Muhammad Zaman asks students in his biomedical engineering honors course Insight and Invention why the symbol of cancer is the crab. A student in the back raises his hand. “Didn’t some Greek guy come up with it?” Indeed, says Zaman, Hippocrates first observed that tumors grow in the shape of a crab.
But there’s another possibility as well, he says. Crabs scuttle back and forth, frontwards and backwards, seeming equally agile in all directions. In the Middle Ages, doctors noted that cancer too was capable of spreading in all directions, just as a crab can move any which way. Historians theorize that it’s the growth of a tumor, rather than its shape, that gave rise to the name of the disease. “Doesn’t sound that profound to me,” says Zaman with a laugh. “But names stick.”
The College of Engineering associate professor of biomedical engineering has spent the last decade trying to understand this peculiar hallmark of cancer: why and how do cancer cells move from one part of the body to another? “When we think about cells in the body, except for blood cells, we don’t think about them moving,” he says. “Two places that cancers tend to move most are lymph nodes and lungs—why? Liver cells don’t move to the lungs, but liver cancer cells sometimes move to the lungs—why? What is different about them?”
It is this ability to move—or metastasize—that makes cancer so deadly. According to the National Cancer Institute, while doctors can cure some types of metastatic cancer, the majority remain untreatable. In fact, most people who die of cancer die not of the primary tumor, but of metastatic disease. Historically, scientists trying to understand metastatic cancer have focused primarily on genes, but Zaman—a biomedical engineer, rather than a biologist—is trying a different tactic. His lab focuses on the physical and mechanical properties of cancer cells, to see if this can explain their growth and movement, then tries to model this behavior in a computer program. It’s a radically different path for exploring cancer, one that only a handful of labs are following.
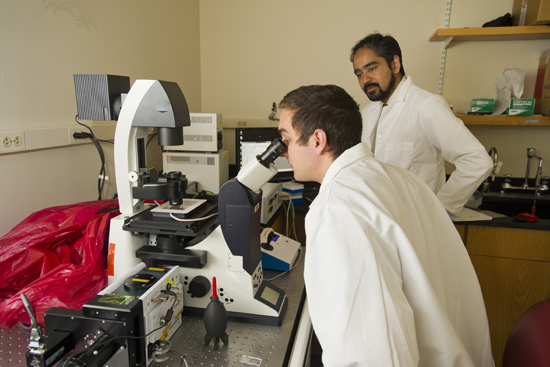
He hopes that this fundamental work will lead to a deeper understanding of cancer and point to possible treatments. “Once we understand the movement,” he says, “we can come up with treatment to stop the movement.”
Zaman, who holds a PhD in chemistry from the University of Chicago, began his cancer research at the crossroads of biology and engineering, as a postdoctoral fellow with a dual posting at MIT’s bioengineering department and the independent Whitehead Institute for Biomedical Research, which is affiliated with MIT. He had no formal training in biology, had never used a microscope, and didn’t even realize that cells moved. But he was intrigued by complex biological systems, and cancer is one of the thorniest.
At the time, most understanding of cancer cell migration came from two-dimensional models: taking cancer cells, putting them on a glass plate, and watching them under the microscope. When cells are grown this way, however, “there are really no mechanical properties to study,” explains Brian Fallica (ENG’14), a PhD candidate in Zaman’s lab. “But in a 3-D environment they behave like totally different cells.” It wasn’t until the late 1990s that scientists began to appreciate how much cancer cells interact with their environment, and thus realize the importance of using more realistic models. Zaman’s task at MIT was to create three-dimensional systems for growing cancer cells, and the computational framework to study them. “It’s a dynamic process,” he says. “If two different people have different environments around a tumor, drugs will react with that cancer differently.” The trick, he says, is understanding not just how, but how much.
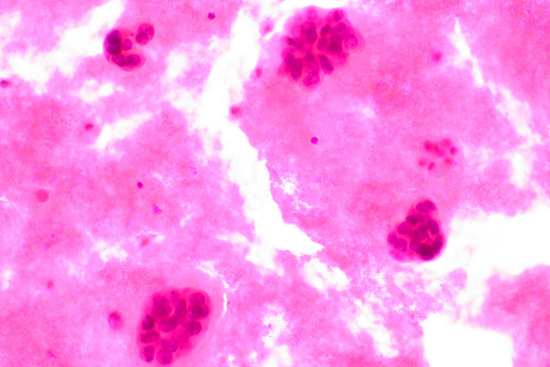
Squishier than normal cells
Zaman’s lab focuses on two major strands of research: the physical and mechanical properties of cancer cells themselves, and the properties of the scaffolds on which they grow. One strange thing about cancer cells, for instance, is that they are squishier than normal cells. Zaman’s group found that this squishiness not only speeds their growth, but also extends their lives—a double whammy that may trigger rapid tumor growth.
To study this peculiar characteristic, the team produced a 3-D computer model that represents each cell as a liquid core encased by a round elastic shell. Then they programmed the individual cells to live, die, or divide based on rules gathered from laboratory observations of tumor cells.
In the computer program, the researchers created simulated tissue composed exclusively of hard-shelled, healthy cells, and then introduced a small number of squishy mutant cancer cells. When the mutants multiplied to eight, they suddenly began to divide at a much higher rate than normal cells. This phenomenon, argue the researchers, represents the emergence of a tumor. Thus, they speculate, a cluster of at least eight soft mutant cells is needed to overcome the resistance of neighboring normal cells so that the mutants can stretch, divide rapidly, and begin to spread throughout the body. The research was published this year in the online edition of the American Physical Society journal Physical Review Letters.
“This study focused only on the first step in tumor growth,” Zaman says. “Our next step is to set up computational experiments to determine what leads tumors to metastasize. Our computer simulations will also allow us to model breast, prostate, and other cancers—even different stages within those diseases—much more efficiently than in laboratory experiments.”
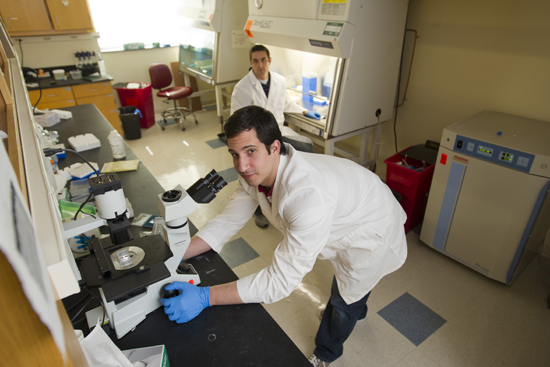
Another group in Zaman’s lab is studying how the mechanical properties of the environment affect how cancer cells grow, spread, and respond to drugs. In the lab, researchers created tiny three-dimensional collagen scaffolds, some stiffer than others. They seeded the scaffolds with cancer cells at different states of development, let them grow, and treated them with chemotherapy. They found, surprisingly, that cancer cells react completely differently to the chemotherapy, depending on the stiffness of the scaffold. In the stiffer scaffolds, chemotherapy drugs hardly had any effect at all, even though the cancer cells were genetically identical. This mimics real-life cancer, where the stiffer, harder tumors are often more difficult to treat. “What was surprising was how stark the difference was,” says Fallica. “The scaffolds were not terribly harder or terribly softer—there was maybe a 10 or 15 percent difference. But that tiny difference led to a 50 percent reduction in cell life.”
The team feeds all the data from their experiments into their ever-growing computer program for analysis. They then use the model to predict and test what they see in the actual cells. It’s a give-and-take between the researchers and the computer, with the program growing more sophisticated as the team gathers more data. In the end, the work could lead to several different applications. Zaman envisions more sophisticated computer models that would allow physicians to input genetic and environmental data for a particular patient to determine what type of drug therapy might work best. Or his research may lead to better scaffolds that could be used in high-throughput drug screening. Or it may lead to new drugs that can thwart difficult environmental hurdles.
“We’re looking at multiple lines of research, some of which are very basic, and some of which are very applied,” says Zaman. His team of about 10 researchers includes only one biologist, and the rest come from various engineering backgrounds. For a cancer biology lab, it’s an uncommon mix, but one that’s leading to uncommon discoveries.
Tomorrow, in the final installment of our “BU Takes On Cancer” series, efforts to prevent breast cancer from metastasizing.
Barbara Moran (COM’96) is a science writer in Brookline, Mass. She can be reached through her website WrittenByBarbaraMoran.com.
Comments & Discussion
Boston University moderates comments to facilitate an informed, substantive, civil conversation. Abusive, profane, self-promotional, misleading, incoherent or off-topic comments will be rejected. Moderators are staffed during regular business hours (EST) and can only accept comments written in English. Statistics or facts must include a citation or a link to the citation.